Most of us take bodily movement for granted. But, in fact, it is a very complex collaboration of many different systems—the long nerves of the pyramidal system, the fine-tuning of the extrapyramidal system and constant sensory feedback. The partnership of nerve and muscle occurs at the neuromuscular junction (NMJ). Recent research shows that that the orchestration of the process that builds a NMJ is extremely complex with elaborate cross talk between the neuron, the muscle and a special glial cell—the peri synaptic Schwann cell (PSC). The complexity of the glia neuromuscular junction, in its final arrangement, includes a perfect alignment of neurotransmitter release sites, clusters of receptors on the muscle and glia processes.
Motor neurons grow huge lengths to reach their final targets. In the adult, motor neurons extend from the spinal cord to the toe with axons as long as a yard. Axons are guided to their exact positions by elaborate signaling from glia and the extracellular space. The final NMJ appears simple, but its formation requires elaborate back and forth signaling. This communication tunes the junction for the precise amount of neurotransmission to meet the muscles’ capacity.
It was previously thought that the pre and postsynaptic cells mature independently, but now it is known that maturation occurs with interaction with all three cells.
Neuro Muscular Junction
The unique synapse between a neuron and a muscle cell is called the neuromuscular junction. The last section of the axon as it approaches the muscle fiber has no myelin. It stops 30nm from the double-layered lipid muscle membrane, called the sarcolemma. It is in this space between the tip of the axon and the muscle membrane where the neuromuscular junction is built. The sarcolemma has an outer coating with a basement membrane of collagen and laminen, which make a scaffold to hold the muscle fibers. Proteins across the membrane connect the inside of the cell’s scaffold and the outside of the cell that connects with the junction.
The space between the nerve and the sarcolemma is called the synaptic cleft, where signaling takes place between the two cells plus the glia, to built the junction. Indentations in the sarcolemma increase the surface area of the cleft and are called post-junctional folds. They become the motor endplate. Receptors for acetylcholine (AChRs) are in these folds with a density of 10,000 receptors per micrometer.
The axon side of the synapse has bulges, called terminal boutons, which align with the post junctional folds. The axon’s terminal boutons have machinery similar to other neurons called active zones containing vesicles of neurotransmitters. Acetylcholine is released by a complex mechanism into the cleft, where sacs merge with the membrane of the axon.
Like other synapses in the nervous system, there are many scaffolding molecules that hold the correct locations of both the nerve and muscle together so that the neurotransmitter flows from the active zone to the cluster of receptors. Unique proteins on the muscle side anchor the receptors. Another critical molecule, muscle specific kinase (MuSK), is an enzyme that adds a phosphate molecule triggering molecular signaling cascades. MuSK and acetylcholine receptors are held in place by scaffolding molecules dystrophin and rapsyn. Another critical molecule to stabilize this entire process is called agrin, which is a proteoglycan—a protein with sugars attached—that interacts with MuSK.
The muscle cell has a distinctive endoplasmic reticulum called the sarcoplasmic reticulum (SR). The SR has unique proteins along the membranes producing molecules to store and pump calcium. It stores calcium in special compartments, which is then released when the muscle is stimulated, coupling the excitation signal from the axon and the contraction of the muscle.
Motor Neurons
NMJs start very early in the fetus after the axon is guided to its muscle fiber. In the spinal cord, columns of motor neurons form. These columns are necessary for the neurons to travel to their correct locations. The axons are guided by signals along the path and timed to meet the muscle in the correct order for development. The axon forms active zones and the muscle forms clusters of receptors.
When the axons arrive, the muscle already has clusters of receptors for acetylcholine (AChR). These early clusters are not the final positioning of the NMJ. When the nerve contacts the muscle, it starts sending excess acetylcholine stimulating the immature NJMs with many neurons attached and large motor units. These junctions are over populated by nerve attachments and maturation of the synapse involves pruning all extra nerve fibers attempting to join the NMJ. Later, with maturation, groups of motor units work together to move muscle groups.
This early NMJ is not able to produce efficient muscle contractions. Movement requires maturation of the NMJ.
Postsynaptic Muscle
Maturation of the muscle connection involves many different changes in the synapse compartment. There are increased number of receptors that are altered in many ways for more stability, increased density and specific formations. For the clusters to form, the proteoglycan agrin is necessary.
The neuron, the muscle and the Schwann cell can make many different types of agrin through RNA alternative splicing. Only very specific versions cause the needed receptor clusters on the postsynaptic muscle through complex signaling cascades of molecules, including extra cellular proteins in the extra cellular matrix (see post on Extra Cellular Matrix) Agrin is secreted into the extracellular space where it is cut by enzymes.
Another complex process removes receptors that are in the wrong places, or are too numerous. When excess acetylcholine is secreted, this stops the creation of more receptors and, in fact, stimulates receptors to be taken back into the cell by endocytosis (sacs). More acetylcholine, also, decreases clustering at one site and increases it at others. When nerve muscle begins to contact the muscle, the neuron secretes agrin to increase clusters. Before the receptors are stabilized, acetylcholine secretion disrupts the receptor formations. It is MuSK and rapsyn that stabilize the receptors working against forces that disrupt them.
Neuronal Presynaptic Maturation
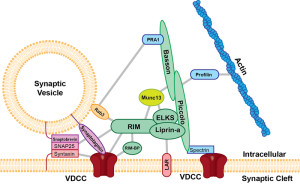
Through elaborate signaling, nerve axon boutons mature by having more active zones and bulges, called ramifications, that align with the post junctional clefts. As neurons mature, they produce active zones, with many associated proteins and more processes. Active zones are very complex structures with a structure of many large molecules (see picture). For this process, new types of calcium channels are produced. Critical to the success of the NMJ is that only one neuron remains as the contact for that muscle.
Special Laminins Are Necessary
Special glycoproteins called laminins are important for the final development of the pre synaptic terminal for the NJM. Laminins, while being structural molecules in the basal membrane are, also, very active in all processes of cellular migration and adhesion. They are secreted by all cells into the extra cellular space, as well as making special structures in each tissue.
Laminins in the basal membrane are complex molecules with three different subunits. While they are used in many different cells, at the NMJ they come from the muscle. Laminins help build more active zones, better clusters of synaptic vesicles, and provides generally better synapse function. The Schwann cells have processes that latch onto the calcium channels using laminin. A series of different calcium channels (N, P and Q types) are used at different stages of development. Large numbers of different laminins align the pre synaptic neuron and the postsynaptic muscle cell in different ways.
There are many different signals and growth factors that are necessary for this maturation, such as fibroblast growth factors, (FGF 7,10 and 22). Collagens (more than twenty kinds) are also, necessary for this complex process. These different molecules have to act in sequence—different FGFs, then laminins and last collagens.
Glial cells are critical to this entire process. Glial cell derived neurotrophic factor (GDNF) along with BDNF are both critical for the creation of the NMJ. GDNF comes from muscle and Schwann Cells and is necessary for the neuron to survive. BDNF is first in a precursor form that is, then, cut to form BDNF. The pro form stops synapses and the full BDNF form is necessary to stimulate synapses. Therefore control over this cutting is critical for the NMJ process.
Neurons, Muscles and Glia Must Signal in Proper Sequence
The large number of different factors and signals have to occur as if in a symphony—at the exact time in the process. The laminins coordinate the junction’s maturation and provide the proper structure for function.
- Laminins of beta1 type bind to integrin to increase clustering of ACh receptors. Laminins with alpha4 or 5 stabilize ACh receptors in the membrane bringing the enzyme necessary to break down the neurotransmitter.
- Laminin 521 inhibits Schwann cells from putting processes into the synapse, where they block transmission.
- LRP4 with agrin, also, works on both pre and post structures and stimulates appropriate ACh receptor clusters, while it is aligns the active zones and the clusters. (top of 708 picture Synapse formation of NMJ
Complex Glia Role in the NMJ
The special glia Schwann cells that surround the synapse—the peri synaptic Schwann cells (PSC)—are both dependent for survival on factors put out by the nerve and muscle fiber, but also provide necessary help for the survival of both. Multiple nerve cells make contact at first and, then, the glial cells’ processes kill them unless they retract. The glia are part of the initial creation of the synapse, but are, also, the critical factor determining which neuron will remain. But, the process of determining the final axon that will be part of the NMJ is, in fact, extremely complex.
The glia cells that surround the NMJ synapse come from stem cells and migrate with the axons to the muscles. When they are at the NMJ, they change shape producing many branches and have decreased mobility. Glia compete with each other for space around the synapse structure as it is built. They prune synapses by phagocytosis (eating them). They express receptors that can tell if the neuron is transmitting or not.
When maturation of the synapse occurs, the NMJ can operate, but it continues to change over a long period from the glia activity. There are many processes that continue to hone its structure and efficiency. When activity starts, all three of the cells are affected.
A critical change is the reduction in the number of connections of nerves with the muscle. At first each muscle fiber has several nerves attached in synapses. Finally, only one nerve connects with the muscle fiber cell.
Neurons Battle for their NMJ
A previous post discussed whether competition among neurons stimulates production of synapses as well as their demise. In fact, in many situations neurons behave as individuals, without a clear rule. Some compete, but others clearly do not. But, at the NMJ, competition is critical to determine which neuronal connection survives. Also, competition occurs when there is nerve damage and the NMJ is re built. But, the competition is not simple and the outcome is never completely clear.
Competition of multiple neurons touching one muscle fiber starts in the embryo and continues after birth. Neurons that are pruned gradually are removed from the region that they are signaling. Other neurons, then, move into the vacated muscle region. The mechanism of retreat for the neuron is in the form of a retraction bulb, which is then eaten by the glia cell.
There does not seem to be one nerve that has the inside track to win this battle, but rather, they actually fight in many different ways to take over each other’s regions. The fight can be ongoing with territory going back and forth between two neurons until one is defeated. A neuron with a smaller territory at the beginning can win. Strangely, even neurons that have much smaller terminals or have already created a retraction bulb can turn the battle around and win. The outcome of the fight cannot be determined by external observation of the structures. Rather, it depends on the complex function of the action zones and receptor clusters.
The patterns of axon activity, also, are important. At first, various neurons have synchronous timing patterns. But, then during competition, they are uncoupled and eventually are completely asynchronous and independent. Experimentally maintaining synchrony, continues the competition longer, while causing asynchrony furthers separation and an end of the competition. When the firing is completely independent, then mechanisms on the postsynaptic structures can focus on one or the other competing neuron.
If one small region of receptor clusters is blocked then the nerve at that region pulls away from the synapse. But if the entire region is blocked it doesn’t pull away. The neuron decides when to pull away. The more active a neuron is, the more it will fire in a way that is not in rhythm with other neurons, which are firing less often. Both the rhythms and the spatial patterns determine the winners and losers.
There are many other complex factors involved in the competition. When there is disruption in one connection, a competing synapse is re built to occupy more territory. Feedback to the glia and the nerve increases the strength of the receptor regions. Pro-BDNF causes negative outcomes and BDNF rewards the signaling terminal. Another factor is transforming growth factor beta1 (TGFbeta1) from the glia cells around the synapse. It increases the clusters by stimulating agrin.
In this competition, constant remodeling of the NMJ occurs. When nerves are pruned, other terminals are either built up or decreased. When a region is left open, the cell moves or removes the clusters of aligned receptors. The endplate changes shape from looking like an oval to a pretzel in this process.
Complex Behavior of the Glia Schwann Cells
As retraction of an axon occurs, glia cells eat them. When eating axon retractions, glia accumulate sacs of debris (axosomes), which are specialized lysosomes. At the beginning of the NMJ development, the Schwann cells cover all of the nerve terminals without choosing the winner. However, as the competition proceeds, they send out processes that interrupt some connections. There is a question whether in this process glia are competing with nerves to be near the clusters of receptors, because glia space their own receptors to be line with each of the competing neuron endings.
Glia then start eating some of the nerve terminals, which makes regions on the muscle vacant. Other nerves, then, take over vacant territory. Glia work with agrin to cut unstable receptors, altering the shape of the endplate to look like a pretzel. Some think that glia eliminate some of the axon endings, helping the eventual winners. To do this, glia must be able to measure activity and timing patterns. They monitor all of the properties of the terminals and send feedback that moves the competition in one direction.
Once the final choice is made the glia take over maintenance of the NMJ. The final coordination is very complex involving positioning hundreds of active zones and receptor clusters. Glia regulate receptor clustering, prune axon retraction bulbs and maintain the synapse, much as the astrocyte does in the brain.
After Injury NMJ Rebuilds
Unlike many parts of the brain, the nerves in the peripheral body can dramatically regenerate after damage. In fact, when a nerve is damaged, the entire nerve, even that part beyond the damage, stays active for some time before re building occurs.
The process depends on the size of the region beyond the injury. First, the blood brain barrier alters to allow more immune cells to enter the region. Then the axon is taken apart at the same time there are an increase of Schwann cells. Glia invade the synapse; macrophages come to eat up material; and the structure of the surrounding extracellular matrix changes. Rather than forming scar tissue, the entire environment is maintained as one that can later accept electrical signals. The Schwann cells build cellular bridges near the neuronal endplate and the receptor clusters. The entire structure including the glia cells continue changing throughout this period to adapt to the level of electrical signaling.
Then the re building occurs. The new axon grows further than the previous neuromuscular junction along the bridges formed by glial cells. Multiple connections are formed, as before the competition. Then new competition occurs in the same way as the previous NMJ.
There are many places where this complex process can go awry. Antibodies to proteins in the endplate cause myasthenia gravis. Many other diseases are caused by other details in the mechanism such as the acetylcholine receptors, lipoproteins and other enzymes.
Complexity of the Glial Neuromuscular Junction
Like so many processes, the creation of the glia neuromuscular junction, critical for all movement, is extremely complex using very elaborate signaling (see post on The Language of the Cell). Although the end result of the process appears to be simple—the alignment of the active zones of the neuron with the clusters of receptors in the muscle—the process is, in fact, totally dependent on extremely detailed communication that must occur in a very precise order. The final structure, also, cannot be attained without the Schwann cell surrounding and maintaining the synapse, as the astrocytes do in the brain. Without these special Schwann cells and elaborate communication between all three cells, there would be no movement.
Where is the direction for this extremely complex process? The three different types of cells appear to act as individuals but work together to form the structure. Direction has to appear for all three cells at the same time, since the NMJ is formed by a large number of back and forth interactions between all three. Also, the timing of each interaction is critical for the end result.
Is this, another, demonstration of mind interacting and directing cells and molecules?