Microbes demonstrate incredible sophistication in making effector molecules that have multi-layered effects. A previous post described how some of these newly created proteins are the exact shapes that alter epigenetic tags in host cells. They produce very particular genetic changes influencing cascades in the nucleus, mitochondria and Golgi that make life easier for an invading microbe. Just as microbes manipulate these vastly complex multi level processes, the secretory machines they build to send their proteins are just as remarkably complex. Some bacteria produce multiple different complex secretory weapons consisting of hundreds of interlocking large proteins. This post describes bacterial secretion systems that are both critical means of warfare and communication.
Microbes Produce Many Secretion Systems
In the battle against much larger and more complex cells, microbes have built these complex weapons to inject toxins, to send material for biofilms and to send genetic information to other comrades. Many different microbes build these extremely complex secretory systems and six basic ones have recently been described in Gram negative bacteria. There are many more in other bacteria. They are, somewhat, similar to a syringe or a phage virus (see post), but built out of a large number of interlocking proteins with multiple layers of complexity. How tiny microbes have developed such advanced weapons is not clear, but they help to stay competitive with the vastly larger and more complex eukaryote cell.
This post will describe six of these complex secretion systems developed by Gram-negative bacteria, one from mycobacteria (tuberculosis), and several others using different mechanisms with chaperone molecules and unique amyloid type molecules called curly. All of these systems secrete small and large molecules, including DNA, RNA and proteins. Microbes use them for all kinds of activity, such as attacking the host, sticking to membranes and survival against the immune attacks form the cell. Some materials are injected into another cell (eukaryote or microbe), some are injected into the space between cells and some stay with the bacteria on their outer membrane (OM).
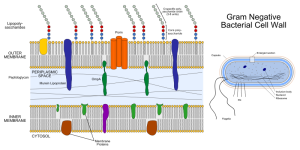
Many bacteria have two membranes—the inner (IM) and outer (OM)—and a space between called the periplasm (differentiated from the cytoplasm inside the cell). Most have one mechanism that shoots unfolded molecules across both membranes. T2SS and pillus and curli types use two steps for the inner and outer membranes and can send folded or partially folded large molecules. Sending fully folded proteins can be done by a specialized system called the TAT (twin arginine translocation system). Another system is used inside eukaryote cells through the ER membrane (SecYeg translocon). All of these systems need to be specifically activated and triggered. It is possible that adhesion molecules can trigger them.
Five types go across both inner and outer membranes—type I secretion system (T1SS), T2SS, T3SS, T4SS and T6SS in the dangerous Gram-negative bacteria. Mycobacteria such as tuberculosis have T7SS. T5SS only goes across the outer membrane as well as two types that form a small structure on the outside membrane consisting of a pillus made of special curli molecules.
This post will describe the vast complexity of these many different secretory weapons.
Microbes Attacks are Multi Dimensional
A previous post documented the complexity of microbe attacks on human cells and specifically the organelles. What is hard to understand is that recent research finds that microbes can alter specific epigenetic tags on histone molecules and DNA. There are now forty different epigenetic tags known that either increase the use of a particular gene or decrease it. Microbes alter these tags to amplify or stop a specific gene that is involved in a particular function as part of a large cascade of molecules. They, also, create new proteins that are close to the human versions, but specifically alter the cascades.
Proteins work through their unique shapes that are determined by the amino acid sequence and ultimately the DNA code and alternate messenger RNA splicing. The largest supercomputers cannot figure out how proteins will fold based on an amino acid sequence, yet microbes can. They design particular enzymes that will alter the epigenetic tags as well. Add to this complexity, the vast sophistication of these secretory systems, it is hard to understand how a small unicellular creature can know how to do all of this.
Type 1 Secretory System
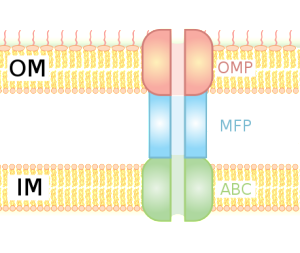
Bacteria that use T1SS systems send various proteins from inside the bacteria to the extracellular fluid. Many of them are related to gathering food, such as enzymes attracting metals. Also, a large number cause damage to other cells, such as producing holes in the membranes.
There are a large number of different types of pumps embedded in membranes that bacteria use for these systems. Pumps are large proteins that provide active transport for materials. They need energy to operate, either high-energy phosphates or electrical gradients. There are, in fact, at least three different pump proteins that all must be working together for these efflux pumps to work.
One particular type of pump that is similar to T1SS is called the RND efflux pumps (for resistance nodulation division). This particular pump is involved in pumping out many powerful antibiotics.
For survival, it is critical for bacteria to become resistant to multiple drugs. They capture materials that can hurt the bacteria and pump it out of the cell, maintaining bacterial resistance to antibiotics. Both RND and T1SS combine three pumps together, including the inner membrane component (IMC), an adaptor protein called the membrane fusion protein (MFP) and the outer protein, which forms a channel. There are multiple kinds of energy attachments, and each have multiple complex parts, which fit and work together. Many of these use ATP as the energy source and the transports vary in structure with the use of the energy alternately facing into the bacteria and then outward
RND uses a proton gradient to combine the material to the pump molecules. A group of molecules cycle through three states where the material is first bound, then transported and then released. These are, also, similar to some of the proteins that viruses use to inject materials. There are many different variations of the structure to accommodate different materials.
The pump has one thin channel, which opens when the inner component combines with the fusion protein at the membrane. The exact mechanism is not known, but recent crystal images of the protein structures have helped to understand it. When material binds to the inner component, energy alters the shapes and it is transported between the inner and outer membranes. Another mechanism uses the proton transport to move it to the middle periplasm region. This triggers a mechanism that opens the outer membrane channel and the release.
Type II Secretion System
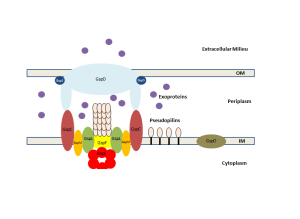
T2SS secretion system is used by many friendly and dangerous bacteria to secrete folded proteins to the extracellular space. It sends enzymes and toxins that are critical for survival of the bacteria. This system has more than a dozen components that make a secretion pathway in many bacteria (e.g., E. Coli, V Cholerae, P aeruginosa).
There are four basic parts. The first is an outer membrane complex. The second is a unique structure that looks like a pillus but is in the periplasm (called the pseudopillus) and is made from a variety of large proteins that look like the materials that make the pillus (the real pillus are the well known string like appendages that stick out from the outer membrane of the bacteria). The five large proteins of the pseudopillus are called pseudophillins and they connect the internal and external membrane complexes. .
The third part is the internal membrane platform and the fourth is an enzyme that attaches high-energy phosphates to the machinery. It is not totally clear how these all fit together.
The outer membrane complex is extremely complex with many components, some similar to phage viruses. There are many different proteins that form a channel through the outer membrane, looking like a closed cylinder.
The internal membrane platform has four large interlocking proteins, one of them connected to the periplasm between the two membranes connecting the inner platform and the outer complex. A six-sided ring shaped set of molecules connects the high-energy enzyme to the inner platform.
Unfolded proteins are transported to the periplasm and then pushed through the outer cylinder like a piston using the pseudopillus.
Type III Secretion Systems
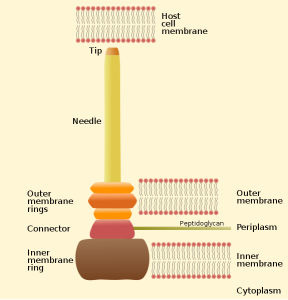
These are critical machinery for many important Gram-negative bacteria like Salmonella, Shigella, Yerinia, Pseudomonas and toxic E. coli. They send effector proteins into eukaryote cells or to the extracellular space. A previous post showed how these effectors subvert the nucleus, ER, Golgi and the mitochondria.
One important example of T3SS is built by Salmonella. It is a large structure made from more than 25 unique interacting proteins that goes across both membranes. It functions like a huge syringe injecting into the eukaryote cell or the extra cellular space. It is called a needle complex or injectisome. There are two main subunits, one appears as rings that are stacked on top of each other and the other is a needle that goes to outside the bacteria. There is, also, a large base associated with many proteins involved in gathering the material for the structure. The base has two rings connected into the inner membrane. There are many other proteins that hold the rings in the periplasm and outer membrane.
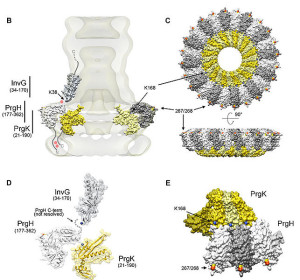
The needle is a long channel, 70nm by 10 nm wide, with a helical structure that goes into the eukaryote cell. It is narrow and can only send unfolded proteins as effectors. When contact is made with the eukaryote cell, special proteins make a hole in the host membrane. Then signals activate the transport of the material. There are chaperone molecules that are essential in this process to coordinate all of the activity. The chaperones keep the effector in the correct state to be transported and keep the machinery stable during the process. At the base of the needle there are special proteins that sort molecules to be transported. The transport is fueled by ATP high-energy particles.
Type IV Secretion System
These are systems that can send DNA and are among the most common in nature. They are involved in spreading plasmids ( small particles of DNA) that have special genes for resistance to antibiotics. They are important for many important bacterial functions used by Heliobacter pylori, E. coli, pertussis and Legionella.
T4SS are built from 12 proteins that form a scaffold, a pump mechanism and a pilus that extends from the bacteria. The pilus extends from the membrane after an enzyme makes a hole for it. It uses three enzymes that make high energy ATP. It has all the same elements as a two part inner membrane complex and then a stalk that creates a core in the outer membrane. The core is made form many complex interacting proteins. There are two layers for the outer and inner membrane with a connecting platform. The total structure is very complex made from hundreds of proteins and the full structure is not known currently. It, also, uses pilli that connect with other cells to transfer DNA and provides adhesion.
Type VI Secretion System
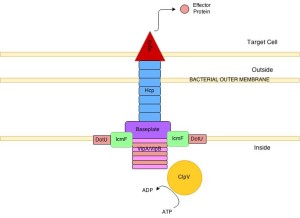
This type goes across the inner and outer membrane and is important for many toxins, both against other bacteria and the eukaryote cell. The cholera type is the most studied. It has a inner membrane complex and a tail complex similar to phage viruses. The tail contracts and is anchored to a membrane complex. It has a long tube that goes far into the bacteria. It forms on a base much like the phage. It has a special platform that is used to assemble the tube, which consists of six sided rings stacked on top of each other.
The structure has been observed as highly dynamic and changeable in its formation. Sheaths first form a large structure almost the size of the diameter of the bacteria then forcefully contract. After this, they come apart and re assemble. The rings have a cog like appearance. The core is built from four protein β sheets with additional stabilizing material. It is not clear how it contracts after the first large structure is formed, but it appears to be responding to a signal and similar to the way phage viruses operate. The molecules to be transported are gathered together and then with one contraction multiple effectors are sent. The machinery is taken apart with the help of ATP from several enzymes. This creates many usable parts for the next event.
Type V Single Membrane System
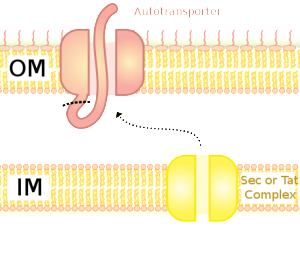
This system is unusual in that the tube for transport and the material transported combine into one large molecule, which transports itself—called the auto transporter. This large molecule made of multiple peptides secretes itself through the outer membrane. It is used to send materials to make a biofilm, to attach to other surfaces and cells and to send toxins. This system uses helper molecules like the chaperone systems (T2SS) to get the unfolded molecule through the inner membrane to the periplasm. Once in the periplasm, part of the large molecule sticks through the outer membrane and part sits in the periplasm. The barrel is formed from β sheets, creating a space to send the molecule out of the outer membrane. It is not powered by protons or ATP but by the force of the folding of the molecule.
There are five different types of T5SS. The most well known is called Va and starts by transport through the inner membrane into the periplasm. Chaperone molecules keep the molecule to be transported in the unfolded state. This chaperone, operating as an enzyme, helps connect the molecules into the form of the auto-transporter. This is sent in the direction of the complex in the outer membrane made of multiple complex proteins and multiple β barrel molecules consisting of four different lipoproteins.
It is not exactly known how the molecule is sent through this outer membrane barrel. The force seems to come from forming a β helix right at the pore in the outer membrane. It appears to thread itself like a screw out of the bacteria. Just as it leaves, the shapes change and an enzyme cuts off one part of the molecule, which goes into the extracellular space. Very recently several large complexes have been discovered that might better explain how this works (translocation and assembly module TAM).
Chaperone Usher Pathway
This cascade of molecules helps create the out pouching from the bacteria called pili or fimbriae. These appendages are made up of a particular protein called curli that is a unique form of amyloid. These curli molecules are vital for many aspects of bacterial function including adhesion, invasion, aggregation and biofilms. They stimulate inflammation. Pili are involved in the T1SS and other specialized forms.
Pili have rod structures smade up of a helix with hundreds of molecules and it has a tip that is flexible and, also, made of many subunits. The many different subunits that are vital for identification of the bacteria in order to have relations with other bacteria and colonies.
To make a pilus, all of the unfolded subunits are secreted into the periplasm through the inner membrane. In the periplasm, these subunits are directed by chaperone molecules to fold and the combined molecule of the chaperone and the subunit are transported to a site where the pilus is being built. A protein enzyme in the outer membrane called the usher builds the pilus through combining (polymerizing) the subunits into the pilus structure. These subunits have structures similar to immunoglobuilins and are made of multiple parts that are specially connected to form the unique structures needed. The part of the chaperone is separated by another special process called the donor strand exchange and it goes back to find another subunit.
The usher protein is, also, very complex with 24 components of a barrel pore that is plugged until it is used to build the pilus. It has special grooves for the chaperones to be separated. Usher proteins are first built in another complex process with an initiation complex and elongation complex. Temporary plugs are taken out and a working plug is put in. When the pili is built and the plug taken out, it is moved into place.
Curli molecules build biofilms to protect bacteria. There are several major subunits that are sent through the outer membrane unfolded and then built into fibers in conjunction with other smaller subunits. It forms the typical β-amyloid sheets (of other amyloids in humans). As with everything else, getting the curli proteins out of the membrane is not simple and several proteins come together with a lipoprotein transporter complex. First a smaller structure is built in the periplasm, but the passage way is narrow, so only unfolded extended molecules can pass through. The process seems to use a diffusion entropy gradient as the power source.
Type VII Secretion Systems in Mycobacteria
This type is specialized to mycobacteria, such as tuberculosis, and are used for their toxins. Mycobacteria have a unique membrane structure, consisting of an inner plasma membrane, a periplasmic space with the molecules pettidoglycan and galactan, and an outer membrane that is unsual being very thick with a special lipid membrane called the mycomembrane.
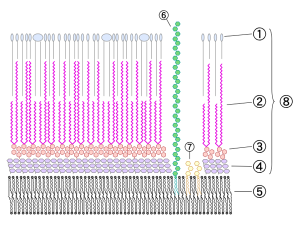
This same structure has now been found in listeria, and staph aureus. There is a central channel made of many proteins at the inner membrane. It has an enzyme for supplies of energy through ATP. This enzyme, also, helps connect the structures and process material. There are proteins that attach to the material to be ejected. The exact mechanism has not been found yet because of the very difficult outer membrane.
Similarities and Differences Between Different Secretion Systems
There are similarities between various systems and unique differences. T2SS and T3SS have the same ringed channel called secretin. But, there are many variations even within the same types. T4SS core outer membrane complexes are somewhat similar. Some have symmetry based on various numbers of molecules—some with 15 molecules, some 12, and some 14. Several varieties have totally unique components.
How each microbe can design unique complex molecules that interact in the proper way with these vastly complex processes is not known. There is a lot that is not known and some bacteria are difficult to study. Many form the same barrels in the outer membrane. There are many unique ATPase enzymes for each type. T2SS, T3SS and T6SS each have one unique enzyme. T4SS uses three completely different enzymes at the same time. One of these makes two copies at the same time that work together.
Antibiotics that Attack Secretion Systems
Antibiotics attack secretion systems. Because there are so many different machines involved, it is difficult to create drugs to counter them. Some bacteria have multiple different kinds of secretion systems at the same. It has been thought that they might use these as signaling devices. With multi drug resistance new drugs are needed.
These secretion systems are vital to the bacterial attack. Research has found several molecules that attack the pilus systems. Ongoing research attempts to prevent chaperones from operating, as well as the polymerization. Curli structures are, also, being attacked. Several new drug compounds stop T3SS from operating in aeruginosa, typhimurium, Chlamydia, and Tersinia. Attack on Brucella is through T4SS.
Intelligent Microbe Secretory Weapons
Secretory systems are extremely complex and sophisticated machines that involve the intricate combination and timing of hundreds of molecules, all in dynamic interactions. These secretion machines are weapons, essential to invade other organisms and communication devices where bacteria stay connected with other comrades.
But, how can one small bacterial cell know that it needs to create a unique protein with a precise code and shape and, then, build a complex unique device to inject it into the other cell in order to alter the second cell’s functions, which provide a living space for the microbe in one of its organelles. These uniquely created proteins can alter the epigenetic tags on DNA and histones to manipulate specific molecules related to the cell’s elaborate function. Remarkably, many microbes build multiple different fantastically complex secretion systems and use them all in different ways.
It is absurd to think that this occurs randomly. Doesn’t the microbe need to have some way of knowing what needs to be built and what its uniquely produced effector molecules will do in the distant host cell.
How can anyone not think that these microbes exhibit extremely intelligent behavior?