Even with intense study for decades, the way that neurons release neurotransmitters so rapidly and continuously is still quite mysterious. The machinery for this variable release of large amounts of vesicles in milliseconds is still being debated. It probably involves multiple different mechanisms. Studies of energy use show that while the brain uses 20% of all of the body’s energy (being 3% of the total mass), most of this energy is consumed by the extremely complex vesicle process at the pre synaptic membrane. It is quite remarkable that such a complex rapidly repeating process can occur so efficiently.
Exocytosis is the general mechanism whereby sacs both merge with and separate from the membrane. For this process to work rapidly, the sacs are filled with neurotransmitters and then released through the membrane. The process must not disrupt the cell’s membrane. Vesicles, or their molecules, are then retrieved and recycled to be used again with the same membrane. The synaptic vesicles (SVs) are merged with the membrane through a mechanism that uses calcium signals.
Many Problems in Understanding Vesicle Release
There are many reasons why we don’t yet fully understand how this complex process really works. First, it is impossible for the neuron to make enough vesicles at the cell body and transport them on microtubules the long distance to the end of the axon. It would take too long for instantaneous responses needed. Secondly, if the vesicles merge with the membrane and are not immediately taken out and recycled, the membrane would be changing shape constantly and become unusable. The membrane tension would be off and the structures that hold the synapse together would be misaligned. Therefore, a very complex recycling mechanism, or mechanisms, operates, but is so complex that it is still not fully deciphered.
Another issue is the time scale involved. Early studies showed perhaps 10 seconds for the membrane to be recycled after a vesicle release. Recent research is more consistent with the realm of a second. Most recently, an extremely fast mechanism appears to be approximately 20 microseconds—vastly more rapid. Part of the problem is the incompleteness of each experimental technique. Some techniques can pick up movement and others pick up specific vesicle protein material left in the membrane. Some operate in seconds, others milliseconds.
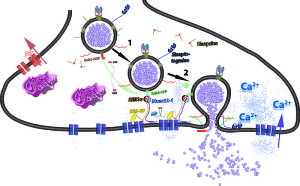
Other research points to the fact that the endocytosis is variable and is related to the intensity of the signal and the importance of the signal. There could be multiple mechanisms working at the same terminal for different types of signals. Calcium levels could stimulate various different mechanisms. Also, the frequency of the firing and the amount of material being secreted could stimulate different mechanisms. Recent study of bipolar cells in the retina shows at least two different mechanisms stimulated by different calcium levels. They each use different materials.
Another difficulty is that different types and sizes of synapses require different types of vesicles and different amounts of neurotransmitters. Although most vesicles are the same size, there are exceptions. Synapses have very different patterns of activity and the amount of neurotransmitter used. One unique very large well-known synapse is called the Calyx of Held, which fires hundreds of times per second with a great number of unique synaptic vesicles. The vesicle has to be taken from the membrane and rebuilt with the proper size and composition.
Four Different Mechanisms
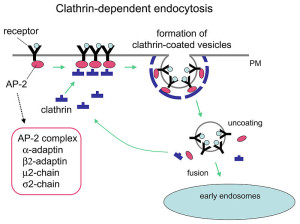
Release of the neurotransmitter vesicles was discovered fifty years ago. They were visualized thirty years ago. The earliest mechanism discovered was clathrin-mediated endocytosis (CME). This is a very complex process where a scaffold structure is built for the vesicle and the membrane.
A second mechanism was discovered more recently called “kiss and run,” which is basically the vesicle fusing and then recycling with a temporary pore in the membrane. Kiss and run may only use a very temporary fusion with the membrane. This could mean the vesicle remains intact to be used again immediately. Recently, there are many very advanced techniques studying this question and it still remains mysterious. Some research is consistent with a real fusion where the proteins of the membrane and the vesicle combine. These proteins, already in the membrane, can then be used again for other vesicles. Along with this model, there are clusters of these proteins in the membrane.
There are now four different retrieval mechanisms described. Kiss and run and clathrin related CME were mentioned already. Two other new versions are the very fast mechanism and bulk endocytosis.
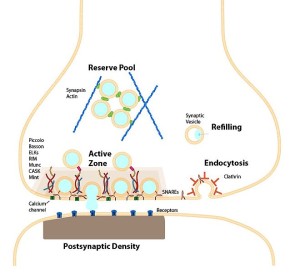
These all occur at or near a region of the pre synaptic membrane called the active zone. The active zone is a section of the out pouching at the end of the neuron before the synapse called a bouton. The active zone includes a region of the neuronal membrane and has a large complex of proteins called the cytomatrix at the active zone or the CAZ.
When viewed under the electron microscope, CAZ appears as a very dark dense region just below the membrane. These proteins create a scaffold that holds synaptic vesicles ready to be released. This very complex scaffold allows for a very reliable mechanism that responds to the arrival of the electric action potential after it has travelled down the length of the axon.
The active zone is juxtaposed across from another unique structure—the postsynaptic density or PSD. The PSD is a very large and complex structure with a thousand interlocking protein molecules in the dendrite of the receiving neuron. Both of these massive and complicated structures have many different variations in different animals and have unique proteins in each region of the human brain. The PSD was discussed in a post on How Do Dendrites Make Decisions.
Kiss and Run
With the kiss and run mechanism, opening of a pore in the membrane is enough to secrete the contents of the synaptic vesicle without other structures. The vesicle that has contacted and covered the fusion pore in the membrane then separates from the membrane and it is used again immediately by being filled with more neurotransmitters.
Kiss and run operates in the active zone. In this mechanism, there is an opening of a hole or pore in the membrane and then rapid closure with the vesicle retaining its shape. This method would not intermingle protein material of any type and would be easier to recycle. This would, also, give a fairly rapid recycling, which is very necessary for many active synapses. Kiss and run has been found in endocrine cells and possibly in neurons. It is not clear how it can avoid the vesicle collapsing.
Clathrin Mechanisms
The now “classical” type of vesicle secretion is based on clathrin or CME (the oldest known mechanism). A pore is formed in the membrane where the synaptic vesicle completely fuses with the cell’s membrane. The material that makes the synaptic vesicle is then taken up with the formation of the clathrin scaffold coating the vesicle. Then, this vesicle is uncoated—that is, the clathrin structure is removed—and the vesicle goes back into the pool of vesicles waiting to be filled with neurotransmitters and then secreted. This is an elaborate and complex procedure that is very slow and operates of the time period of seconds, even tens of seconds. It is not clear how such a complex mechanism can work in rapid neuronal transmission. But, it appears to be part of several mechanisms.
CME is utilized for transfer of vesicles between cells such as endocytic transport of proteins and lipids, which is much slower. See previous posts for a discussion of this communication technique between cells. However, research is increasingly consistent with the fact that it is, somehow, used in synaptic vesicles, as well, since the materials used in CME are found in synapses. Several of these proteins, such as dynamin and endophilin are used by other methods as well.
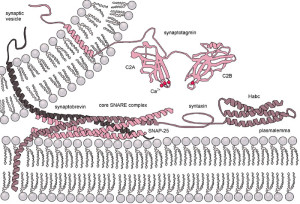
Clathrin coats the vesicles with a polyhedral lattice structure with three heavy chains and one light chain. There are special complex proteins that assemble this coating for the vesicle, called assembly protein or AP. These adaptor molecules connect clathrin coat to the membrane via phospholipids. This very complex mechanism, also, uses proteins that go through the membrane. Another part of the mechanism involves clathrin coated pits (CCP), which use a wide range of different proteins in different versions of this very complex process.
Perhaps the most crucial element is SNARE complexes—soluble NSF attachment protein receptors. SNARE structures are drivers of the release of the synaptic vesicles. The process involves coating the vesicle, fission and then un coating. This system is so complex it is not likely that it can be accomplished quickly. Because of this, it can only be used in certain circumstances. High frequency neurons in the hippocampus were not affected by eliminating this mechanism. (See post on endosome communication between neurons and glial cells).
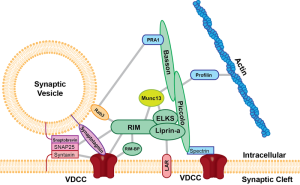
When the action potential comes to the end of the axon, the neurotransmitters have been loaded and ready to be sent in milliseconds. These are ready to fuse with the membrane through SNARE, which is attached to the membrane and the vesicles. There are a large number of different proteins that are part of this process including synaptobrevin, syntaxin and SNAP25. The proteins assemble in a particular way which pulls the vesicle closer to the membrane waiting for the calcium signal to fuse. Calcium is triggered by a special sensor. The membrane is disrupted with acid from the proteins sandwiched with phospholipids and calcium. With the membrane disrupted, it produces a go signal for the fusion. Complexin regulates energy barrier for the disruption.
The New Ultrafast Mechanism
In this form of endocytosis, synaptic vesicles are completely merged into the membrane for the release of the neurotransmitters. Ultra fast is new model where the vesicles are recovered and sent to merge with a large endosome vesicle where another vesicle is manufactured with the same CME process. Then, 10 milliseconds later, markings appear near the active zone at a different place than where the vesicle fused with the membrane. These indents mark an area that is equal to four vesicles.
The ultra fast process doesn’t appear to need clathrin mechanisms. Both actin and dynamin seem to be involved in this extremely rapid mysterious process. It is not clear how the vesicle can merge with the membrane so rapidly. Other studies show much more time is needed for this to occur. There are other dynamics of the membrane tension near the active zone. The fusion seems to create a tension in another area near the active zone where the new vesicle material is taken in by invaginations.
In the ultrafast mechanism membranes of the synaptic vesicles are very rapidly retrieved. This occurs after a single action potential or more. The invaginations are not in the active zone, but at its side. While it is not totally clear what occurs, the synaptic vesicles are reformed from the vesicles or from other very large vesicles in the cell that are similar to vacuoles. This second process can be complex and involved in the slow clathrin mechanism despite being part of the ultrafast process.
Bulk Endocytosis
The fourth mechanism is called bulk endocytosis. Strong tetanic or chemical stimulations cause the appearance of large bulk endocytic structures (very large vesicles like vacuoles) which are subsequently resolved into smaller synaptic vesicles via clathrin-dependent and/or clathrin-independent mechanisms.
Bulk endocytosis produces very large folds of the membrane on the far side of the active zone from the fusion. This appears to involve a large amount of special proteins and very active firing of the neurotransmitter vesicles. A large piece of membrane forms a very large empty vesicle, called a vacuole. This large vacuole then makes smaller synaptic vesicles from the material. It is in some ways similar to ultrafast, but different scale and speed. Clathrin is possibly involved as well as dynamin. Dynamin seems to be necessary for the fusion.
Synaptic Vesicles are A Precise Size
One unusual aspect of this entire process is that almost all of the vesicles are the same exact size and shape (with very few exeptions). They have a precise protein makeup, which is necessary to allow the fusion to occur without damaging the membrane. One vesicle has about 600 large proteins that span across the vesicle membrane surface. These proteins make up a quarter of the surface area. Several of these proteins are critical to the process and each vesicle only has a small number of these unique proteins. The proteins have to be maintained in the process of reconstruction of the vesicle. When proteins are not exact, an elaborate sorting occurs to provide the exact number. It is very surprising that this can all happen so quickly, without obvious direction.
Clathrin, and especially its adaptor proteins, seems to be somehow involved in many of the processes. Research has shown that if there are abnormal adaptors then the synaptic vesicle is not made the proper size. The adaptor proteins last for days and are used in multiple cycles of the fusion with the membrane and reconstitution of the vesicle.
Confusion has been increased by recent studies that show processes not involving clathrin. Another set of proteins is found that re form the synaptic vesicles called AP-3. There is some evidence that particular proteins act with very specific sub populations of the vesicles even in one synapse.
Aside from neurotransmitters, endocytosis is a general cellular phenomenon. A previous post described multiple ways that cells communicate using various vesicles and with connecting nanotubes (cytonemes). The transporting vesicles are critical ways that cells send nutrients, proteins and genetic material between cells such as neurons and glia. These endocytic pathways are critical to capture and kill microbes using lysosomes (vesicles with enzymes that destroy microbes and other cellular waste products). It is reasonable that some of the same machinery would be involved with synaptic vesicles.
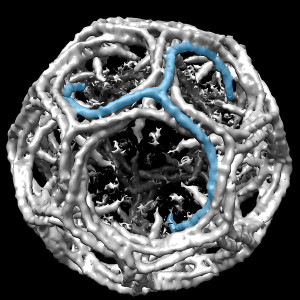
Removal of used vesicles at the membrane is necessary to maintain the active zone’s activity of storing, docking and rapidly releasing vesicles. To accomplish this, specific membrane tension has to be maintained. There is limited space at the active zone for storing vesicles to be used. After release, the reuptake of the material or the vesicle is a critical function to keep the active zone functioning. The general endocytic pathways may help in this retrieval process.
Clathrin processes certainly are able to help separate material and re constitute vesicles to then be docked at the active zone. There is speculation that a process de activates clathrin when very rapid turnover is needed. In this situation the vesicle is kept whole as in kiss and run. In other circumstances, clathrin will help with the slower process of rebuilding and separating the exact types of vesicles needed.
Many Unique Mechanisms in Various Brain Regions
The pool of vesicles readied to be released is called the readily releasable pool (RRP). Recent research shows that there is great variation in determining which of these will be released, but the reasons are not clear.
Each vesicle is filled with thousands of molecules of neurotransmitters in seconds. This process uses energy from a proton gradient. It has not yet been understood how molecules with charges can be so closely contained with the vesicle being stable and electrically neutral. Recent research shows that different neurotransmitters, such as glutamate and GABA use different mechanisms—glutamate uses ions in the transport and GABA an exchange for protons alone.
When the electrical action potential arrives near the presynaptic terminal, voltage changes open calcium channels and calcium ions rush in and diffuse to sensors to trigger release of the vesicles. These sensors trigger release of particular vesicles. Calcium channels and sensors can be close together or not and exist in many different 3 dimensional patterns. And there are different nano mechanisms for this process to occur. Some sensors are right at the mouth of the calcium channel. Other patterns include many different channels that have complex summations and triggers. This complexity is similar to the electric channels noted in the post on how dendrites compute.
There are many different versions of sensors and channels now discovered. Most are very specific single setups with some overlapping multiple channels and sensors. Some have unique geometrical arrangements.
- Sensory cells with unique ribbon release synapses use rapid and sustained release.
In the auditory hair cell, extremely rapid (less than a millisecond) pulses open only a small number of accurately defined available calcium channels.
- In the eye’s rod cell a tonic depolarization with a broad range for faint and varying light
- The neuromuscular endplate uses a very specific 23 nm distance between the sensor and the channel
- Fast neuron-to-neuron synapses have many different patterns.
- Amacrine retinal neuron has a particular calcium channel (CaV1) with a variable mechanism where the distant channels are used less as the eye opens.
- Cortical pyramidal neurons use many overlapping domains of sensors and channels.
- Hippocampus neurons have very specific domains with channel and sensor 20 nm apart and mossy fibers in the CA3 have complex overlapping domains.
While this amazingly elaborate process works, it is easy to understand problems that can arise that can cause brain disease. Genetic abnormalities in proteins related to synaptic vesicles have been noted in neurodegenerative diseases such as familial early onset Alzheimer’s and in some forms of ADHD.
Mysterious Pre Synaptic Vesicles
There are many unanswered questions to this improbable rapid process of neurotransmitter release in vesicles. It uses most of the brain’s energy, which is 20% of all human energy. One important question is how the neuron keeps track of how many vesicles have been released in order to re cycle them? Proteins left over at the breakup might signal the number. Somehow, the tension in the membrane is measured and monitored as well. The precisely timed release is triggered by many specific calcium sensors and channels.
Mental processes stimulate these massive, complex, energy sapping, repetitive synaptic vesicle mechanisms. Where is the direction for the extremely complex processes described, occurring simultaneously all over the brain in milliseconds?
Synaptic vesicles are another example of fantastically complex systems that are essential for the human brain. How can anyone think this is a random process? How can this be directed without mind interacting with the entire brain, specific neurons, vesicles, active zones, and molecules?